In the realm of tech innovation, the next big thing might just be growing beneath our feet. The The latest research from the Unconventional Computing Lab (University of the West of England) led by Neil Phillips in cooperation with Roshan Weerasekera, Nic Roberts, Antoni Gandia and Andy Adamatzky delved into the fascinating world of fungi, revealing the potential for mycelium-bound composites and fungal fruiting bodies to become key players in the development of sustainable, bio-hybrid computing systems.
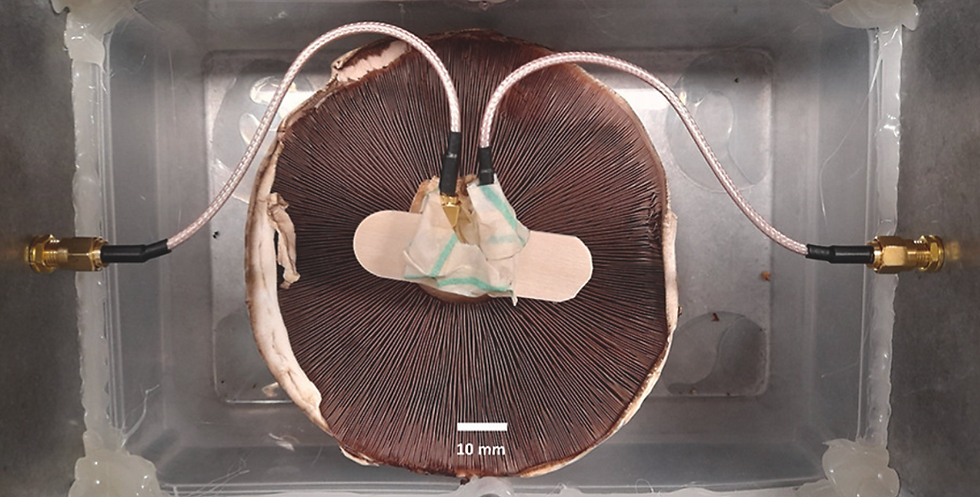
Mycelium, the intricate network of fungal threads that colonize organic material, is being harnessed to create novel electronic components. These composites, formed by binding lignocellulosic substrates with fungal hyphae, can be moulded into various shapes and sizes. Recent studies from the Bristol, U.K. based laboratory have shown that live fungi within these composites exhibit unique electrical properties, including memfractive and capacitive behaviours. "Memfractive" refers to a material's ability to exhibit a combination of memory and refractive properties. In simpler terms, it's when a material can remember past electrical signals and change how it conducts electricity based on that memory. This makes it useful in creating smart electronic components that can adapt and respond to different inputs over time and positions them as potential candidates for integration into electrical circuits with nonlinear characteristics.
Exploring The Mechanics of Fungal Electronics
The research explored how mycelium-bound composites (materials made by binding plant fibres with fungal threads) and fungal fruiting bodies (the part of the fungus you see above ground) conduct alternating current (AC) electricity across a wide range of frequencies.
Key Findings
Mycelium-bound Composites:
Function: Act like low-pass filters. Think of a low-pass filter as a sieve that lets low-frequency signals pass through while blocking higher frequencies.
Performance: They have an average cut-off point around 500 kHz. Above this point, they start blocking signals more significantly.
Roll-off: They block higher frequencies at a rate of -14 dB/decade. This means that for every tenfold increase in frequency, the signal strength drops significantly.
Pass Band Attenuation: On average, the signal strength drops slightly (by 1 dB) even in the frequencies they let through.
Fungal Fruiting Bodies:
Function: Also act as low-pass filters but with a lower cut-off frequency range (5 kHz to 50 kHz depending on the species).
Performance: They start blocking signals at much lower frequencies compared to the mycelium-bound composites.
Roll-off: They block higher frequencies more aggressively, at rates between -20 dB/decade and -30 dB/decade.
Pass Band Attenuation: On average, the signal strength drops more (by 3 dB) in the frequencies they let through compared to the mycelium-bound composites.
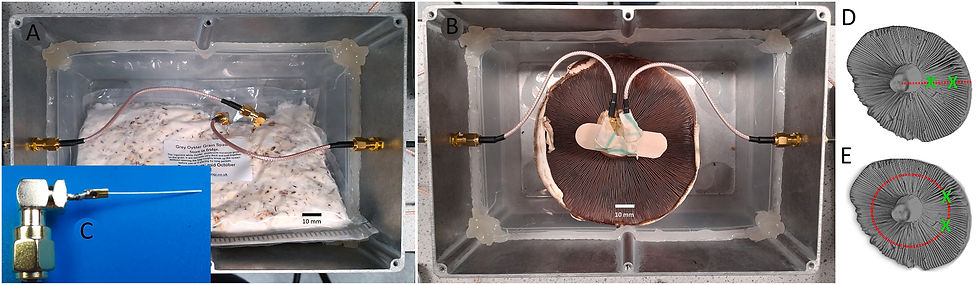
Electrodes inserted into (A) mycelium bound composite (Grey Oyster spawn) in bag, scale bar is 10 mm (B) fruiting body (Agaricus bisporus) inside RF shielded enclosure via coaxial connections, scale bar is 10 mm (C) platinum iridium needle electrode with SMA connector (D) axial alignment of electrodes (marked by ‘X’) in cap of fruiting body (E) radial alignment of electrodes (marked by ‘X’) in cap of fruiting body.
What's Causing This?
The exact reason for how these materials block higher frequencies is not fully understood. However, it is likely related to their high water content, which conducts electricity due to dissolved ions (charged particles in the water).
In simpler terms, both the mycelium-bound composites and fungal fruiting bodies can act like sieves for electrical signals, allowing only certain frequencies to pass through. The mycelium-bound composites can handle higher frequencies before blocking them, while the fruiting bodies start blocking much lower frequencies more strongly. The water inside these structures probably plays a key role in this behaviour.
Beyond the Lab: Ecological and Technological Implications
Understanding the electrical activity of fungi is not just an academic exercise. It has profound ecological implications. Fungi play critical roles in ecosystems, from nutrient cycling to symbiotic relationships with plants. Monitoring their electrical activity can provide insights into their physiological states and environmental responses, which is crucial for ecosystem management and conservation efforts.

Fungal fruiting bodies (A) Agaricus bisporus (Portobello), (B) Pleurotus ostreatus (White Oyster), (C) Hypsizygus tessellatus (White Shimeji), (D) Pleurotus djamor (Pink Oyster), (E) Cantharellus cibarius (Chanterelle), (F) Pleurotus eryngii (King Trumpet), (G) Lentinula edodes (Shiitake).
A Sustainable Future with Fungal Electronics
The potential applications of mycelium-bound composites in analogue computing are vast. These materials could serve as frequency filters in oscillator computing or handle sensory data in edge computing. The integration of living mycelium networks with traditional silicon components could lead to groundbreaking advances in bio-hybrid technologies.
Moreover, fungal electronics offer an environmentally friendly alternative to conventional electronics. They are inherently biocompatible, capable of interfacing with biological systems, and possess self-healing properties due to their natural growth and regeneration processes. This adaptability, combined with their ability to biodegrade, positions fungal electronics as a sustainable solution to the growing problem of electronic waste.
The Path Forward
This research highlights the need for interdisciplinary approaches to fully harness the potential of fungal electronics. By exploring the electrical properties of mycelium-bound composites and fruiting bodies, we not only pave the way for innovative computing technologies but also deepen our understanding of fungi’s ecological roles. Future studies should aim to correlate electrical activity with environmental factors like soil pH, temperature, and moisture levels, further unravelling the ecological significance of fungal electrical behaviour.
The implications of this research suggest that fungi, through their electrical properties, offer a unique perspective on the complex interactions that sustain ecosystems. This could revolutionize agricultural practices, optimize mushroom cultivation, and enhance soil health. As we continue to explore the "mycological iceberg," the fusion of electronics, mycology, and ecology could lead to a future where fungal electronics play a role in sustainable technological innovation.